Why Teach Educational Robotics?
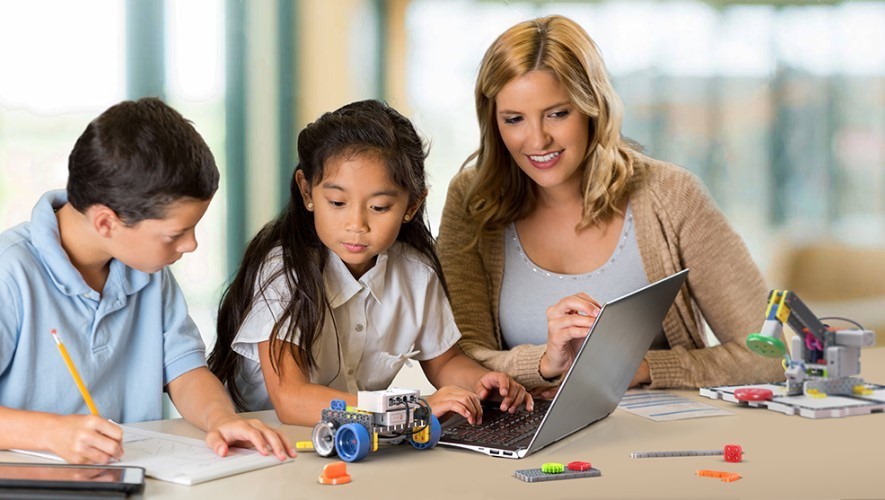
Over the last several years, interest in Educational Robotics has flourished as teachers and schools embrace the potential of robotics to provide hands-on and engaging ways to teach design, engineering and technologyi. Also seen as a way to introduce and incentivize students to pursue careers in Science, Technology, Engineering and Math (STEM) fieldsii, the use of Educational Robotics is now more affordable and robust, thanks to all of the increased attention and investments given to the medium. The resulting technological advances greatly contribute to the accessibility of this tooliii. In fact, robotics are now considered by some to play a similar role in the classroom as computers once did, beginning with the early 90’s and the introduction of the use of CD-ROM’s and Microsoft PowerPoint in classroomsiv.
With Educational Robotics’ growing presence comes important questions. What are the best uses of this new and exciting tool? How can we establish best practices? How do we conceptualize the purpose of Educational Robotics in the classroom? These questions may be more complicated than they seem at first glance. And answering them may first produce more questions than when we began. For example, do students use educational robotics as a medium to display their ideas and thinking, or do students create ideas and thinking by interacting with the medium? Are Educational Robotics a way for students to show their competency, or are they an infrastructure upon which students build new competenciesv? Perhaps considering an aspect of computer use in the classroom can help to shed more light on the topic.
A medium can have a different scope based on its application. Painting can be seen as a medium, one that can be used to paint a fence or the Sistine Chapel. The versatility of computers as a medium has, arguably, even greater enormity; a computer can be used in the classroom with a very limited scope, either as a calculator or as a word processor, yet also seen and embraced as a powerful means of communication all its own. As Mark Guzdial has pointed out, computers can be understood as a modern form of Gutenberg’s printing press vi, and as a way to think about other domains. As such, technologies like computer modeling and algorithms have had a significant impact on our understanding of the fields of math and sciencevii.
What then, is the scope of Educational Robotics? Educational Robotics can be used as pre-built objects that perform very specific tasks, while some Educational Robotics systems allow students to become active participants in designing their learning – as well as creators of computational artifacts, instead of passive users of devices that others have made for themviii. This presents a unique set of opportunities for teachers. Educational Robotics thus becomes a medium providing students the opportunity to exercise their voice and choice in learning and engage them not only in problem solving, but also in problem finding, problem construction, problem analysis, and the planning and monitoring of problem-solving efforts. Educational Robotics then, becomes something much bigger - a medium to prepare students for the complexity of the challenges awaiting them as they prepare for jobs that currently do not existix, and also a way to incorporate other valuable dexterities (e.g. communication and collaboration) belonging to the broader spectrum 21st century skills.
The efforts by schools to implement the medium of Educational Robotics seem to have produced as many manifestations as different motivations driving the initiatives. Some schools use this tool as an integrated part of a stand-alone computer science or STEM course, while other schools use this modern solution to supplement traditional subjects. Still other schools use them as after-school activities that then capitalize on the motivational effects of “gamifying” and competitions to raise student participation and engagement. In the same manner that schools learned not to restrict computers’ use to expensive calculators, the use of Educational Robotics should not be limited by perceived constraints.
Worth exploring in detail are the following uses for Educational Robotics:
- To understand our world
- To teach integrated STEM education in novel ways
- To teach Computational Thinking
- To become comfortable with iteration and learn from failure
- To be exposed to and learn about the jobs of the future
To understand our world
Science is the explanation of the natural world. Students that are scientifically literate are able to understand both the concepts and practices of science. Therefore, teaching students science offers them an opportunity to understand the world they inhabit. This is why high school curricula all over the country includes subjects like Astronomy, Biology and Chemistry. But what of Robotics? Clearly, robots are prevalent in our everyday lives, and that prevalence is increasingx. Improvements in the technology associated with robots, has led to exponential growth of computation power and data storagexi. This has resulted in robots capable of learning and making decisions informed by the experiences of other robots. Robots are no longer machines that perform simple functions. Additionally, the rising demand for robots and robot technology cuts across industries. Yes, factories are homes for many robots, but robots are also now more common in educational and entertainment settings. It is quite possible that in the near future robots are assisting many members of the elderly population live independently in their homes, thus creating a new field of “co-robots.”xii
Schools, rightfully, teach about planets and stars that exist light years away…but not about the technology that many interact with on a daily basis. This is a challenge, but also an opportunity. Education drives science and innovation. The study of Biology continues to lead to better treatments and the eradication of sicknesses and diseasexiii. If Robotics became a core academic subject in our schools, it could potentially have a similar impact.
To teach integrated STEM education in novel ways
Educational researchers suggest that teachers often struggle to make connections across STEM disciplinesxiv. This presents a challenge to schools as the Next Generation Science Standards feature cross-cutting concepts spanning different scientific domains. Therefore, students will have difficulty transferring concepts oftentimes taught in isolation to the integrated context they will see on assessment exams. Another unintended consequence of teaching scientific concepts in isolation, is its tendency to create a learning environment where students become disengaged. The authentic examples they see of science in their daily lives has deep integration across STEM disciplines as opposed to singularity. The goal of STEM education is to help students organize information within and across disciplines, to be able to identify and reason with deep, structural similarities and patterns within this information; the culmination ideally resulting in the ability to apply this organization of knowledge to complex situations and problems in everyday lifexv.
Educational Robotics can help address these challenges by functioning as a facilitator for teachers and schools as they look to organize STEM instruction. Since the scope of Educational Robotics moves far beyond a toy that can be given simple instructions, classrooms that utilize Educational Robotics can offer students robust engineering and programming challenges.
To Teach Computational Thinking
During the past 10 years, Computational Thinking has grown in popularity and inclusion within K-12 classroomsxvii. Computational Thinking is included as part of the Next Generation Science Standards and as an essential part real-world math and science. Computational Thinking is widely considered to be an integral part of any STEM classroom xviii.
"A primary motivation for introducing computational thinking practices into science and mathematics classrooms is the rapidly changing nature of these disciplines as they are practiced in the professional world." (BAILEY BORWEIN 2011; FOST ER 2006; HENDERSON et al. 2007)
"In the last 20 years, nearly every field related to science and mathematics has seen the growth of a computational counterpart." (WEINTROP et al. 2017)
The increase of the popularity of Computational Thinking as a concept, both in and out of schools, has led to schools attempting to find effective tools to integrate and teach computational thinking to their students. A corresponding goal has been to broaden participation in the classes- particularly computer science - that delve heavily into computational thinking; addressing the gender gap in this subject area has also been a consistent goal. Currently, girls account for approximately half of all AP test-takers, but account for only 25% of those taking AP computer science classesxix.
Educational Robotics can be an effective tool to teach computational thinking while also helping to broaden participation goalsxx xxi. Recent advances in Educational Robotics have lowered costs and increased ease of use, making them more accessible to students and progressively turned to as a reliable way to learn abstract STEM concepts. As such, the connection between computer science and robotics is clear; students have the ability to program their robots to perform complex tasks, both in the classroom and on competition fields. While the performance of complex tasks may be the end, the means involve decomposing these tasks into smaller parts and then iteratively building them together to create a solution. In classrooms, the scaffolding of that process is vitally important, and once again, Educational Robotics can be effective in facilitating both the decomposition and scaffolding of complex tasks, As a result, robots can be an effective tool to teach computational thinking, as the initial evidence shows.xxii xxiiiThe effective teaching of computational thinking also results in the ability to apply computational thinking in different domains. The ability to effectively teach generalizable computational thinking skills, while simultaneously offering ways to help diversify the students that enter these fields, makes Educational Robotics a significant contributor to the integration of computational thinking into schools and the Computer Science for All movement.
To Become Comfortable With Iteration and Learn From Failure
Engineering design and the scientific method are related phenomenon, but contain important distinctions. In science, there is an emphasis placed on finding general rules that describe the actions of our world and universe, whereas engineering involves finding solutions to a particular problem that satisfies all of the constraints contained within that problemxxiv. Some have summarized this distinction with the saying “scientists investigate but engineers create” xxv When considering the creative process, we must recognize its often significant dependence upon iteration.
Multiple iterations are crucial to engineering ideas and activities that are designed to achieve certain objectives, whether meeting/exceeding customer expectations or participating in a competitive challenge. The required multiple iterations inherent to Educational Robotics activities have been recognized as capable of maintaining student interest and sustained engagement.xxvi Also, the composition of the robotics kits themselves, with many different pieces that can be quickly assembled and then disassembled, fosters an attitude of iteration. Because multiple iterations often address the important life lesson of “try, try again,” students benefit immensely from learning that “failures” can be embraced as all part of the process. Another broadly applicable lesson borne of a more abstract look at the ancillary benefits of the tool is Educational Robotics’ tendency to present multiple solutions to even the simplest challenges. What could broaden a student’s horizons more than realizing there are indeed multiple solutions to the same problem? We’ve seen this produce interesting benefits: an increased likelihood in students requesting feedback from teachers and a higher likelihood of students understanding what they are learning as important.xxvii The benefits only compound from there - teachers engaging students in this way can lead to higher student self-efficacy, the key element leading to that greater willingness to learn from failure. xxviii
To Be Exposed To and Learn About the Jobs of the Future
Change, our only constant, is no stranger to the nature of work. In 1900, approximately 40% of the American workforce worked on farms. Today that number is only 2%.xxix If that seems too long ago, too far-removed, consider that as recently as 50 years ago, the average worker did not need to read or write during their workday.xxx The tides of today can be epitomized in a widely read and discussed 2013 study by The University of Oxford Department of Engineering Science, which estimates that 47% of current jobs are at risk of being lost to automation.xxxi
An important distinction of the current concerns, as opposed to the normal churn of job destruction and job creation of yesterday, is “job polarization.” The term applies to the hollowing out of employment opportunities, meaning, there is high-demand for high skill and low skill jobs, but opportunities for middle-skilled and middle-wage jobs have declined.xxxii This significant problem can be traced to the automation of routine work, and the answers involve acknowledging the inevitability of automation by working creatively towards augmentation. The businesses riding these wave successfully are those responding with flexibility and fluidity, learning to work with technology rather than running from or rebelling against its daunting presence and impact.xxxiii As educators, it is vital we too respond creatively, searching for innovative solutions to the uncertainty of the future. It is up to primary and secondary education systems to acknowledge the realities on the horizon, and teaching relevant and valuable skills, which in the current case, may mean things computers are simply not good at. These include creativity, interpersonal skills, and problem solving, all skills that can be cultivated be a refined use of Educational Roboticsxxxiv.
Source: VEX Robotics
i Alimisis , Dimitris, editor. Teacher Education on Robotics-Enhanced Constructivist Pedagogical Methods . School of Pedagogical and Technological Education , 2009.
ii iii Eben B. Witherspoon, Ross M. Higashi, Christian D. Schunn, Emily C. Baehr, and Robin Shoop. 2017. Developing Computational Thinking Through a Virtual Robotics Programming Curriculum. ACM Trans. Comput. Educ. 18, 1, Article 4 (October 2017), 20 pages
iv “Computers in the Classroom.” Wikipedia, Wikimedia Foundation, 10 June 2018, en.wikipedia.org/wiki/Computers_in_the_classroom. v David Weintrop and Uri Wilensky. 2017. Comparing Block-Based and Text-Based Programming in High School Computer Science Classrooms. ACM Trans. Comput. Educ. 18, 1, Article 3 (October 2017), 25 pages.
v Guzdial, Mark. Learner-Centered Design of Computing Education: Research on Computing for Everyone. Morgan & Claypool Publishers, 2016. vii Weintrop, D., Beheshti, E., Horn, M. et al. J Sci Educ Technol (2016) 25: 127. https://doi.org/10.1007/s10956- 015-9581-5
viii Martin, F., Mikhak, B., Resnick, M., Silverman, B. and Berg, R. (2000). To Mindstorms and Beyond: Evolution of a Construction Kit for Magical Machines, Morgan Kaufmann Series in Interactive Technologies, Robots for kids: exploring new technologies for learning, Pages: 9 - 33
ix Herold, Benjamin. “The Future of Work Is Uncertain, Schools Should Worry Now.” Education Week, Sept. 2017.
xi Dang, Sanjit. “The Robot Revolution Is Just Beginning.” TechCrunch, TechCrunch, 4 June 2018, techcrunch.com/2018/06/03/the-robot-revolution-is-just-beginning/. xii Johnson, R Colin. “‘Co-Robots’ Help Boost Human Productivity.” Electronic Engineering Times , 12 Aug. 2012.
xiii “5 Groundbreaking Breakthroughs in Biology.” Brainscape Blog, Brainscape Blog, 26 May 2017, www.brainscape.com/blog/2015/06/biology-breakthroughs-and-discoveries/.
xiv Kelly, Todd R, and J. Geoff Knowles. “A Conceptual Framework for Integrated STEM Education.” International Journal of STEM Education , 19 July 2016.
xv Honey, Margaret, et al. STEM Integration in K-12 Education: Status, Prospects, and an Agenda for Research. The National Academies Press, 2014.
xxii Eben B. Witherspoon, Ross M. Higashi, Christian D. Schunn, Emily C. Baehr, and Robin Shoop. 2017.
Developing Computational Thinking Through a Virtual Robotics Programming Curriculum. ACM Trans. Comput.
Educ. 18, 1, Article 4 (October 2017), 20 pages
xxiii Liu, A., Schunn, C. D., Flot, J., & Shoop, R. (2013) The role of physicality in rich programming environments.. Computer Science Education, 23(4), 315-331
xxiv Specialty, School. “The Scientific Method vs. The Engineering Design Process.” Schoolyard, 15 Nov. 2017, blog.schoolspecialty.com scientific-method-vs-engineering-design-process/.
xxv Katehi, Linda P. B., and Greg Pearson. Engineering in K-12 Education: Understanding the Status and Improving
the Prospects. National Academies Press, 2009.
xxvi Silk, E. M., Higashi, R., Shoop, R., & Schunn, C. D. (2010). Designing technology activities that teach mathematics. The Technology Teacher , 69 (4), 21-27
xxvii Marzano, Robert J., Debra Pickering, and Tammy Heflebower. The Highly Engaged Classroom. Bloomington, IN: Marzano Research, 2011. Print.
xxviii Marzano, Robert J., Debra Pickering, and Tammy Heflebower. The Highly Engaged Classroom. Bloomington, IN: Marzano Research, 2011. Print.
xxxiii Raman, Amy BernsteinAnand. “The Great Decoupling: An Interview with Erik Brynjolfsson and Andrew McAfee.” Harvard Business Review, 13 Mar. 2017, hbr.org/2015/06/the-great-decoupling.
xxxiv Raman, Amy BernsteinAnand. “The Great Decoupling: An Interview with Erik Brynjolfsson and Andrew McAfee.” Harvard Business Review, 13 Mar. 2017, hbr.org/2015/06/the-great-decoupling.